The subject of vaccination is fraught with complexity. Scroll through any article discussing the COVID-19 vaccines and you will see terms like 'mRNA', 'viral vectors', and 'lipid nanoparticles'. While mRNA technology is remarkable, many of us are unaware of how the immunizations we received as children work. In the words of Richard Saul Wurman, “You only understand information relative to what you already understand”. Accordingly, in order to fully understand the significance of the latest vaccine technologies, we must understand the nature of pre-existing, licensed vaccines.
“You only understand information relative to what you already understand.” - Richard Saul Wurman
If you’re eager to understand but feel hindered by the complexity of the topic, in this article, I will break down the 5 different types of vaccines (including the hugely talked about mRNA vaccine), in an attempt to demystify this convoluted subject. While I will concede that I love a concise article, the reality is that this is a complex topic with many questions, so I did my best to cover it all. Scroll down to number 5 on the list to read about mRNA vaccines.
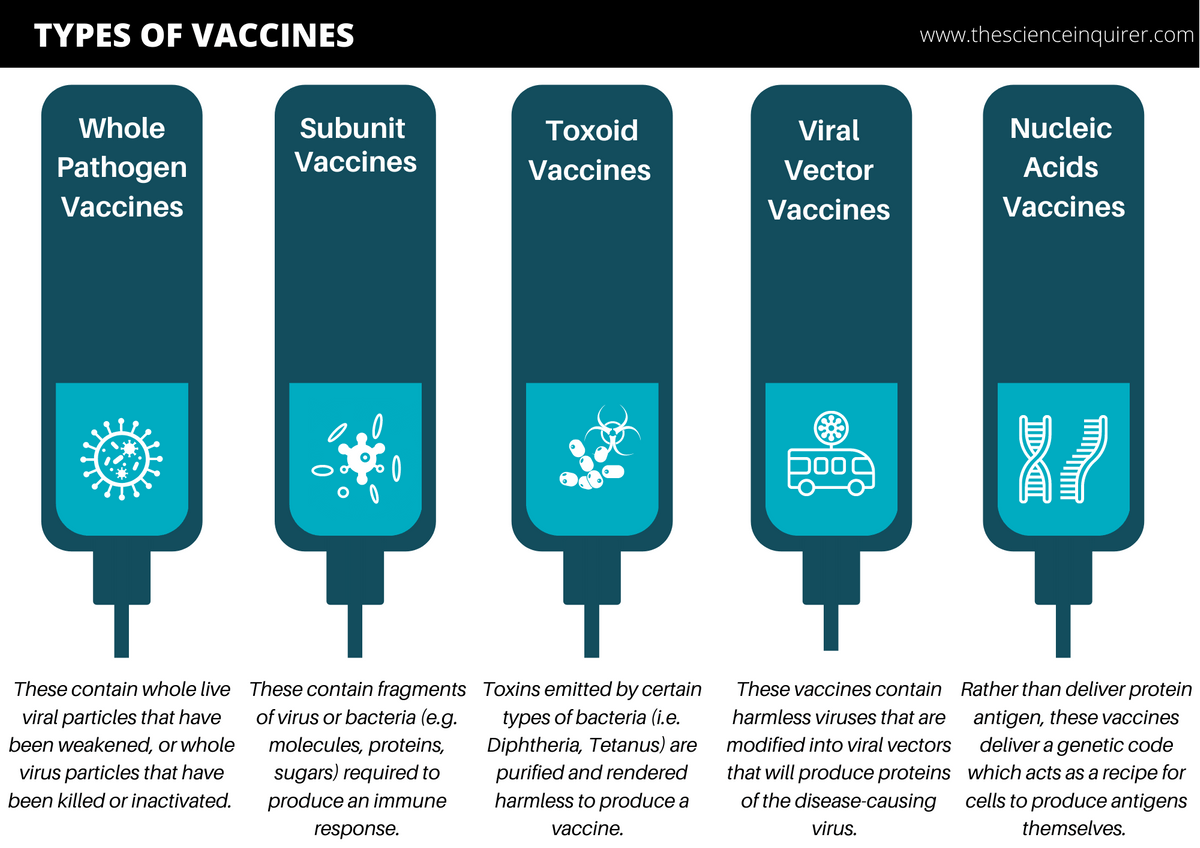
1. Whole Pathogen Vaccines
This is the most well-known type of vaccine in which the entire disease-causing pathogen, or virus, is used in the vaccine to generate an immune response equivalent to a natural infection.1 Since using the whole pathogen in its natural state would cause disease, pathogens in these vaccines are altered to ensure they cause no harm.
There are 2 sub-types:
a) Live-Attenuated (LA) Vaccines
These are vaccines that contain a living but weakened version of the virus.2 The idea here is if a weakened virus is used, it will not create serious disease (i.e. “limited virulence”) in those with healthy immune systems, and will not have the capacity to spread.2 Some of the most effective vaccines in use today are live-attenuated vaccines.3
Examples of licensed live-attenuated vaccines: MMR (Measles, Mumps, Rubella), Chicken Pox (Varicella), Oral Polio (Sabin), Influenza (the seasonal flu nasal spray and the 2009 H1N1 nasal spray), Rotavirus & Yellow Fever.2
Live-attenuated COVID-19 vaccines currently in the development pipeline:
1. COVI-VAC is manufactured by Codagenix Inc., a clinical-stage biotechnology company situated in New York that develops vaccine products. COVI-VAC is "a single-dose, intranasal, live-attenuated vaccine against COVID-19, generated using Codagenix’s proprietary deoptimization technology."74
2. Indian Immunologicals Ltd. (IIL) is the leading manufacturer of veterinary and human biologics in India. In collaboration with Australia's Griffith University, scientists are developing a live-attenuated SARS-COV-2 vaccine.75
How are viruses weakened for LA vaccines?
Typically, a virus is weakened through “serial passage”.4 Serial passage refers to the growth, collection, and re-growth of cells in a vessel in specified growth conditions for many iterations. First, scientists use cells from a different species (for example, chicken embryonic cells in the case of the measles, mumps, and rubella vaccine) and infect them with the virus.5 As the virus adapts to a new environment (i.e. different growth mediums in a new host species), the virus mutates repeatedly to become better adapted to this host, thereby weakening its disease-causing abilities within human cells.4 These weakened “mutant” strains are then selected for use in vaccines as they are significantly less virulent (infectious) and will not cause disease, but instead help the immune system develop protection. Alternatively, a virus can also be weakened by selecting mutant strains that have different growth temperature requirements relative to the natural virus. In summary, a pathogen can be attenuated by growing it in sub-optimal conditions and selecting a strain that has undergone sufficient mutation to render the pathogen harmless once inside a human host.2
What are the advantages of LA vaccines?
- Well-established technology and simple manufacturing process12
- Highly immunogenic: They are highly effective as it activates a broad range of immune responses and best mimics a natural infection.2
- Long-term response: Induces long-lasting and rapid onset immunity.2
- Single dose: A single dose is often sufficient for long-lasting immunity. This reduces the need for booster vaccinations.2
- Adjuvants are not required: Adjuvants are ingredients that help vaccines generate stronger immune responses and antigen stability. Live-attenuated vaccines often contain naturally occurring adjuvants, therefore adjuvants do not need to be added to vaccine formulations.2, 3 An additional advantage of the absence of adjuvants is that non-adjuvanted vaccines tend to elicit fewer local reactions (redness, swelling, and pain at the site of injection), as well as fewer systemic reactions (chills, body aches, and fever).6
What are the disadvantages of LA vaccines?
- Reversion to virulent virus: In rare circumstances, because the virus is still alive, there is a risk of the weakened virus reverting to the virulent form through secondary mutations, thereby causing disease.7
- Culture Contamination: As these vaccines are grown in large culture vessels (a “container” containing living cells with necessary amino acids, minerals, growth factors etc.), there is a risk of contamination during the manufacturing process.1
- Not recommended for pregnant women: When vaccinating pregnant women, there is a theoretical risk of fetal transmission as some live vaccines can pass the placental barrier. That being said, unintentional vaccination of pregnant women with live vaccines is not indicative of pregnancy termination.8
- Not recommended for immunocompromised individuals: Contraindicated in those with congenitally acquired or pharmacologically induced immunodeficient states because there is risk of the weakened virus demonstrating increased virulence causing severe disease.9
- Requires refrigeration to remain immunogenic: Careful storage is required as LA vaccines are temperature sensitive.12
b) Inactivated Vaccines
Inactivated vaccines contain whole viruses which have been killed or modified so that they cannot replicate.1 The virus is typically killed by exposure to a physical or chemical substance which destroys infectivity.10 Although the virus has been inactivated, it still retains the ability to be recognized by the human immune system. In order for inactivated vaccines to be effective, the method of rendering the virus inactive, whether via physical or chemical exposure, must not affect the structure of the key “antigens” on the virus.
Examples of inactivated vaccines: Poliovirus, Cholera, Hepatitis A Virus (HAV), Japanese Encephalitis Virus (JEV), Tick Borne Encephalitis Virus (TBEV), Rabies, Influenza11
Inactivated COVID-19 vaccines either in the development pipeline, or permitted for emergency use:
- CoronaVac is manufactured by Sinovac Biotech, a Chinese biopharmaceutical company. This vaccine is a chemically inactivated, whole-virus preparation that is administered in a two-dose regimen.76
- BBIBP-CorV was created by the Beijing Institute of Biological Products and trials were run by the state-owned Sinopharm, a Chinese pharmaceutical company. Beta-propiolactone was utilized to inactive the virus. It was then mixed with an aluminum-based adjuvant to boost the host immune response.77
- Covaxin is manufactured by Bharat Biotech ICMR, a vaccine & biotherapeutic manufacturer in India. This chemically inactivated, whole-virus preparation is also administered in a two-dose regimen.78
How are viruses inactivated?
Viruses are typically inactivated using chemical substances or exposure to heat.12 Formaldehyde (also referred to as formalin or formol) and beta-propiolactone are most frequently used for inactivated virus preparation. Formaldehyde inactivates viruses by essentially getting the “building blocks” of the virus, such as proteins, to irreversibly bind to each other in unusual ways.10 This inactivates the virus while maintaining the sites that the human immune system will recognize to help produce antibodies.
Heat can also be used to inactivate a virus as it does not alter the genetic code of the virus, but instead damages the outer shell of the virus (known as the capsid).10
That being said, our immune system is not as good at recognizing microorganisms that have been treated by chemical or heat exposure which is why inactivated vaccines are often given in multiple doses, require a booster shot many years later, and/or contain adjuvants.12
Is there formaldehyde in inactivated vaccines?
Yes, however, formaldehyde is diluted during the vaccine manufacturing process and the amount remaining as a result of this process is considered insignificant.14 Interestingly, while most people think of formaldehyde as a man-made chemical, it is found naturally in the body in small quantities.8 It is actually an important metabolic intermediate produced by enzymatic reactions (i.e. one-carbon cycle),10 to help synthesize DNA and amino acids (the building blocks of proteins).15, 16
In fact, the European Food Safety Agency (EFSA) conducted a study and estimated that the human body naturally makes and processes approximately 878 – 1310mg of formaldehyde per kg of body weight per day.17 To put this in perspective, the amount of natural formaldehyde found in a 2-month old baby’s blood is ~1.1mg in total, ten times greater than the (less than) 0.1mg of formaldehyde found in any vaccine.14
Paradoxically, formaldehyde is still toxic in larger quantities and the above information is not intended to negate the fact that chronic exposure to formaldehyde has been linked to cancer; however, relative to the amount of formaldehyde naturally present in the body, the quantity found in some vaccines is too small to pose a safety concern.13, 18
What are the advantages of inactivated vaccines?
- Well-established technology and relatively simple manufacturing process
- No reversion to virulent disease: Since inactivated vaccines do not contain live viruses, they cannot revert back to the original and more severe disease.12
- Safer and more stable than LA vaccines
- Can be administered to the immunocompromised12
- Ease of transportation: Most inactivated vaccines do not require refrigeration and can be easily transported in a freeze-dried form.12
What are the disadvantages of inactivated vaccines?
- Weaker immune response: The immune response is weaker and less comprehensive than the live attenuated (LA) vaccine.
- “Booster” shots may be needed: This helps the recipient of the vaccine maintain immunity.12
- Adjuvants often accompany inactivated vaccines: Adjuvants help the inactivated virus stimulate the immune system.
- Some viruses are not immunogenic after inactivation: This leads to short-lived immunity.12
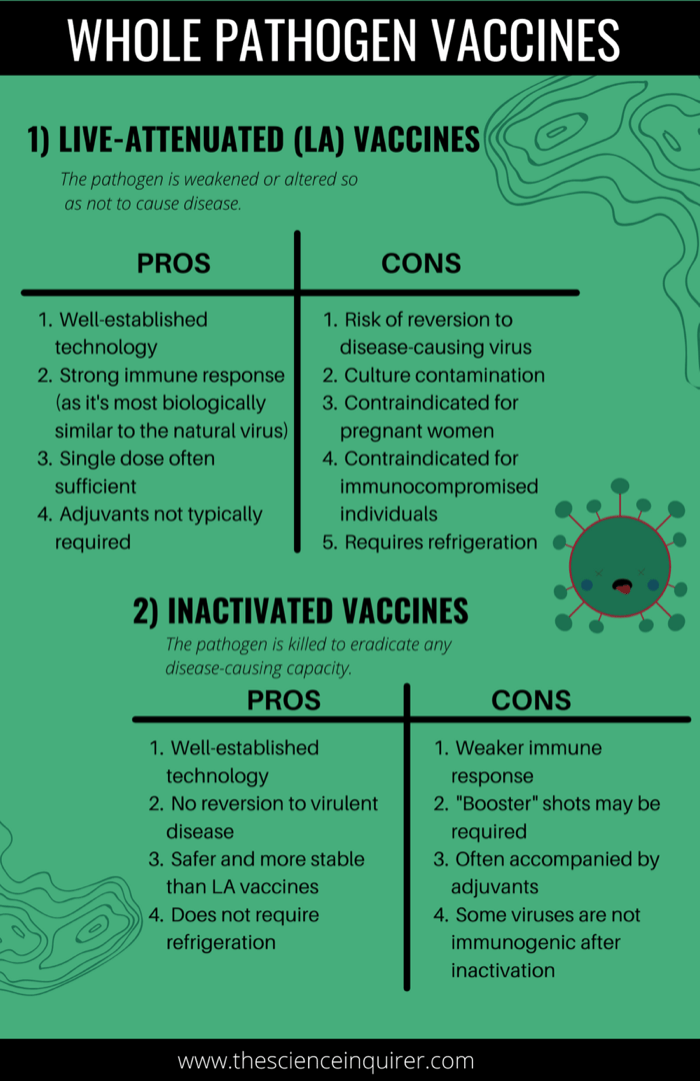
2. Subunit Vaccines
Unlike LA or inactivated vaccines, subunit vaccines do not contain whole pathogen, but instead contain fragments of the virus or bacteria required to produce an immune response.19 This can include single molecules, proteins, or even complex sugar molecules.19 When subunit vaccinations are administered, an immune response focused specifically on these antigen targets is generated.19 As it doesn’t target the entire virus, subunit vaccines do not always elicit a strong, long-lasting immune response relative to LA vaccines.2 This subgroup of vaccines typically requires booster shots and the addition of adjuvants (definition: substances that amplify the immune response).2
There are 5 sub-types listed below:
a) Recombinant Protein Vaccines
Recombinant vaccine technology entails inserting a small segment of DNA which encodes a protein antigen (such as a viral or bacterial surface protein) into “manufacturing” cells.20 These cells are typically bacterial or yeast calls which can produce the protein from the virus.2 This protein is then purified and utilized as the active ingredient in the vaccine.20
Examples of licensed recombinant protein vaccines: Hepatitis B, Human Papilloma Virus (HPV), Meningococcal Bacteria Type B2
b) Polysaccharide Vaccines
Polysaccharide vaccines are a unique type of subunit vaccine that facilitates the development of immunity for a different type of bacteria, sometimes referred to as “encapsulated” pathogens. This subtype of bacteria disguises itself by wearing an outer capsule composed of complex sugars known as “polysaccharides”.21 This outer sugar capsule makes it more challenging for the immune system to recognize antigens. To develop immunity against encapsulated pathogens, you need to train the immune system to mount a response to polysaccharides, rather than proteins. Essentially, polysaccharide vaccines train the immune system to recognize and mount a response against the molecules in the pathogen’s outer capsule.21
Early on in the development of polysaccharide vaccines, it was discovered that it was difficult for a young child’s immature immune system to recognize and respond to the “sugar coat”. Therefore, polysaccharide vaccines are not efficacious in infants and young children under 18-24 months.22 As well, polysaccharide vaccines tend to induce only short-term immunity and repeat doses of these vaccines do not elicit a booster response.22
These types of vaccines have been used to create immunity against the following diseases: Pneumococcal disease, meningococcal disease, Salmonella Typhi22
c) Conjugate Vaccines
Similar to polysaccharide vaccines, conjugate vaccines also elicit a response against molecules in the pathogen’s capsule. Unlike polysaccharide vaccines which induce short-term immunity, conjugate vaccines induce long-term protective immunity, even in infants. Conjugate means ‘connected’. When researchers learned that polysaccharide vaccines were often ineffective in children, they set out to develop a technology that would be effective in infants.2 Scientists later discovered that polysaccharides connected (conjugated) to antigens induced long-term protective immunity, even in children.2 Essentially, conjugate vaccine technology entails connecting the polysaccharide to a carrier protein which helps the immune system recognize the coating and mount an immune response. The majority of conjugate vaccines entail connecting a polysaccharide to a toxoid protein, such as diphtheria or tetanus toxoid protein.2
Examples of licensed conjugate vaccines: Haemophilus influenza type B (Hib) vaccine, Children’s pneumococcal vaccine, MenC vaccine, MenACWY vaccine 2,23
d) Virus-Like Particle (VLP) Vaccines
Virus-like particles are molecules that imitate the structure of authentic native viruses, but lack the viral genome.24 As such, they are not infectious and have the potential to yield safer vaccine candidates. VLPs are comprised of one or more structural proteins that are arranged in several layers. In certain instances , the antigens in a VLP vaccine are the viral structural proteins themselves.25 Alternatively, the outside and inside of VLPs can be modified to enhance their immunogenicity, or to present foreign antigens to the immune system through genetic fusion or chemical conjugation.25 When presented to the host immune system, VLPs can evoke effective immune responses without prompting the side effects of native virus.26
Examples of licensed VLP vaccines used for: Hepatitis B virus, HPV2
e) Outer Membrane Vesicle (OMV) Vaccines
Outer membrane vesicles (OMVs) are protrusions (or ‘blebs’ in cell biology terms) of the outer cell membrane of bacteria which are naturally secreted.27 OMVs are spherical, double layered membrane structures that possess many elements present on the external surface of bacteria.28 These nanostructures serve as a delivery vehicle for DNA, RNA, proteins, and toxins. Interestingly, not only are OMVs the ideal size to be taken up by immune cells, but these bacterial nanoparticles also have the ability to present antigens to induce immune responses.29 Accordingly, OMVs are an intriguing vaccine platform with the added benefit of intrinsic adjuvant capacity.29 OMVs can also be modified so that any potentially toxic antigens are removed, and those antigens that are ideal for the stimulation of an immune response are retained.2 OMV vaccines entail harvesting OMVs from bacteria and are used as the active ingredient in the vaccine.
COVID-19 subunit vaccines in the development pipeline, or permitted for emergency use:
1. NVX-CoV2373 is manufactured by Novavax Inc., an American vaccine development company. This vaccine contains a full-length, prefusion spike protein using recombinant nanoparticle technology and a proprietary adjuvant.79
2. Adaptvac is a "joint-venture between NASDAQ First North listed ExpreS2ion Biotech and University of Copenhagen spin-out NextGen Vaccines".80 The venture is known for its proprietary Virus-Like Participle (VLP) technology. In May 2020, Bavarian Nordic signed an agreement with AdaptVac to license AdaptVac's capsid virus like particle technology for COVID-19 indication.81
These types of vaccines have been used to create immunity against the following diseases: Meningococcal B Vaccine 2
What are the advantages of subunit vaccines?
- Well-established technology relative to newer vaccine technologies
- No living vaccine components: As subunit vaccines contain no live virus, there is no risk of inducing the disease.19
- Often suitable for immunocompromised individuals
- Potential for new vaccines: Opportunity to develop vaccines where traditional approaches have failed (e.g. HIV vaccines)19
- Batch consistency: Produced in a highly differentiated state which improves consistency between batches of manufactured vaccine.19
- Targeted immune responses toward specific microbial or viral determinants.19
- Ease of transportation: Can be freeze-dried allowing non-refrigerated transport & storage.19
What are the disadvantages of subunit vaccines?
- Decreased immunity, limited duration: Subunit vaccines are weaker than traditional whole pathogen vaccines. This is owing to a restricted number of components with the ability to stimulate the immune system.19
- Complex manufacturing: Determination of the optimal “subunit” combination requires more time.
- Addition of adjuvants: To address decreased immunity, the addition of adjuvants is typically required to ensure long-term immunity.19
- Booster shots may be required: Subunit vaccine booster shots may need to be administered at several points in a patient’s life.19
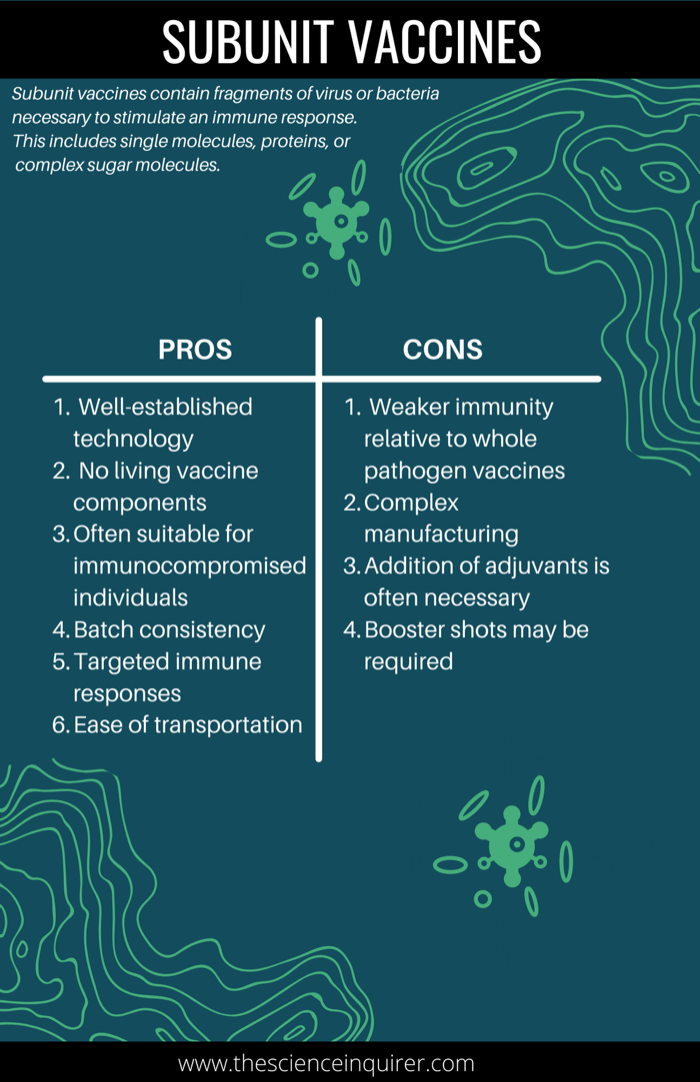
3. Toxoid Vaccines
Some bacteria produce poisonous proteins, or toxins, when they infect the body which cause the primary symptoms of a disease.7 Toxoid vaccines work by protecting the body from the toxins, rather than the pathogen itself. Similar to any other antigen, the immune system also recognizes toxins and launches an immune response. These vaccines are referred to as ‘toxoid’ vaccines rather than toxin vaccines because the toxin has been made harmless to ensure they cannot cause illness. In other words, ‘toxoids’ are inactivated toxins.30 Toxoid vaccines are manufactured by purifying the bacterial toxin.7 The toxicity of the toxin is then suppressed through inactivation with heat or formaldehyde to produce toxoids.7
These types of vaccines have been used to create immunity against the following diseases: Diptheria vaccine, Tetanus vaccine, Pertussis vaccine2
What are the advantages of toxoid vaccines?
- No reversal to pathogenic wild-type virus:
- Vaccine does not cause the disease it is designed to protect from30
- Stable & long-lasting: Less prone to temperature, light and humidity changes30
- Rare local and systemic reactions30
What are the disadvantages of toxoid vaccines?
- Not highly immunogenic on its own so it typically requires adjuvants 30
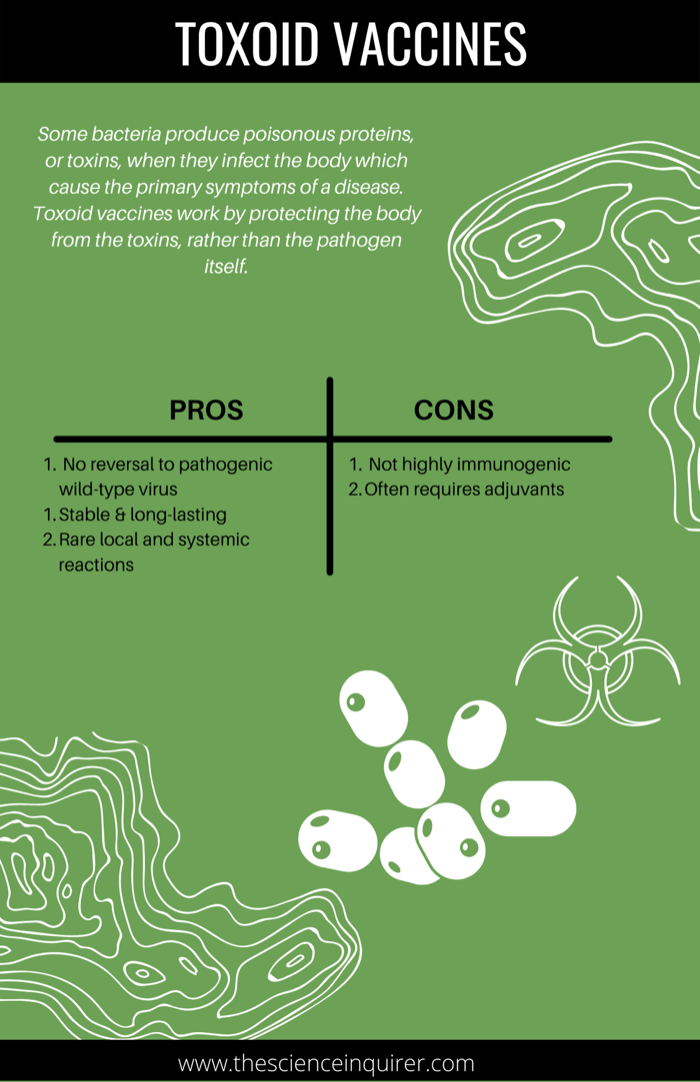
4. Viral Vector Vaccines
Viral vector vaccines are a newer technology which leverage the ability of viruses to infect cells and deliver their genetic material to infected cells.31 This technology entails using harmless viruses as a delivery mechanism, and modifying them to express the genetic code of the target vaccine antigen. When an individual is vaccinated, the harmless virus which expresses the code for the antigen is delivered to cells where the actual protein antigen is produced, thereby stimulating an immune response. The adenovirus (which causes the common cold) is among the most heavily utilized viral vectors for vaccine development.32
There are 2 sub-types of viral vector vaccines:
a) Replicating Vector Vaccines
In addition to delivering the vaccine antigen, replicating viral vectors are still able to produce new viral particles and propagate themselves once infection occurs.33 As with live attenuated vaccines, the integral advantage of this delivery and propagation mechanism is that as a replicating virus it can continuously generate vaccine antigen over a prolonged period of time in comparison to non-replicating vector vaccines.33 A beneficial consequence of this is the provocation of a stronger immune response.33 You may be wondering whether the use of a virus to deliver an antigen is disease-inducing. Generally, harmless viruses or weakened viruses are chosen so that while they “infect” the host, they cannot trigger disease. Nevertheless, a consequence of the occurrence of viral replication is a greater chance of mild adverse reactions to these vaccines. The measles virus and the vesicular stomatitis virus (VSV) are commonly used replicating vectors.32
Examples of licensed replicating vector vaccines: An Ebola vaccine manufactured by Merck & Co. (an American pharmaceutical company) called Ervebo utilizes the vesicular stomatitis virus (VSV) as the vector, and a genetically engineered protein from the Zaire ebolavirus to trigger an immune response.2, 71
b) Non-Replicating Vector Vaccines
Unlike replicating vector vaccines, non-replicating viral vectors do not retain the capacity to generate new viral particles upon delivery of the vaccine antigen to the cell.33 In other words, when they enter cells, they produce vaccine antigen but cannot create new virus particles. These vaccines utilize vectors (carrier viruses) that can induce a host immune response but cannot replicate inside cells. This is attributable to the removal of genes responsible for replication in the lab. The primary advantage of a non-replicating vector is it cannot cause disease, and adverse events associated with replicating vectors are reduced. The drawback of this vaccine subtype is a weaker immune response as the vaccine antigen can only be produced so long as the initial vaccine is still present in cells.
These types of vaccines have been used to create immunity against the following diseases:
1. Ebolavirus: In July 2020, Janssen’s Ebola Vaccine was licensed for use by the European Medicines Agency. It utilizes an adenovirus and a Modified Vaccinia Virus Ankara (MVA) as it’s carriers, or vectors. Both have been genetically modified to ensure they cannot replicate in human cells.72
COVID-19 non-replicating vector vaccines permitted for emergency use:
- The Oxford-AstraZeneca COVID-19 vaccine is a non-replicating vector vaccine has been granted regulatory approved for emergency use in several nations including Australia and the UK, and is under review by Health Canada at present . It utilizes a non-replicating viral vector called ChAdOx1. 2 which is derived from a chimpanzee adenovirus.73
- Sputnik V COVID-19 Vaccine was developed at the Gamaleya National Research Centre for Epidemiology and Microbiology (Moscow, Russia). It uses a recombinant adenovirus approach using adenovirus 26 (Ad26) and adenovirus 5 (Ad5) as vectors which express the SARS-CoV-2 spike protein.82
- Johnson & Johnson JNJ-78436735,
also known as Ad26.COV2.S, is a non-replicating vector vaccine which uses a replication-incompetent adenovirus serotype 26 (Ad26) vector that encodes the full-length SARS-CoV-2 spike protein. It is currently under review by the World Health Organization (WHO).83
What are the advantages of viral vector vaccines?
- Strong immune response: Stimulation of innate immune response.34
- Adjuvant typically not required: Since vector vaccines trigger a strong immune response, adjuvants are not typically required.34 Studies have been conducted with viral vaccines in conjunction with adjuvants but observed no increased immunity.35
- Booster shots not always required: A single dose can be sufficient for protection, particularly in the case of replicating vector vaccines.33 However, in the case of non-replicating viral vector vaccines, booster shots may be needed. This is because vaccine antigen can only remain present so long as the initial vaccine is present in cells (as non-replicating vectors do not produce new viral particles).
* Non-replicating viral vector vaccines tend to have a reduced chance of adverse events relative to replicating vector vaccines
What are the disadvantages of viral vector vaccines?
- Theoretical risk of reversion to wild-type virus: Since the viral vector component of these vaccines are derived from some form of pathogenic virus, whether intrinsically harmless or attenuated, there is a theoretical possibility of reversion to the ‘virulent’ disease.35 That being said, safety testing has indicated that this is unlikely.35
- Previous exposure to the vector can reduce vaccine effectiveness (otherwise referred to as induction of anti-vector immunity): Viral vectors contain their own viral antigens in addition to the vaccine target antigen.36 While this makes the vectors effective at eliciting an immune response, it also makes vectors prone to launching an immune response against themselves. In other words, the immune system would launch an immune response to the vector, as oppose to the antigen. When an individual has pre-existing anti-vector immunity (meaning they have been previously infected, or immunized with the vector virus) this reduces the effectiveness of the vector vaccine. In order to circumvent this issue, scientists have employed strategies such as using strains of adenovirus that are less likely to circulate among humans to ensure an effective immune response against the target antigen is launched. 36
- More complex cell-based manufacturing: Scalability is a bottleneck of viral vector vaccine production as viral vectors are typically grown in cells adhered to a substrate.37 The process of producing a vector vaccine entails several steps and components which augments the risk of contamination. Consequently, frequent testing is required which increases the cost of production.
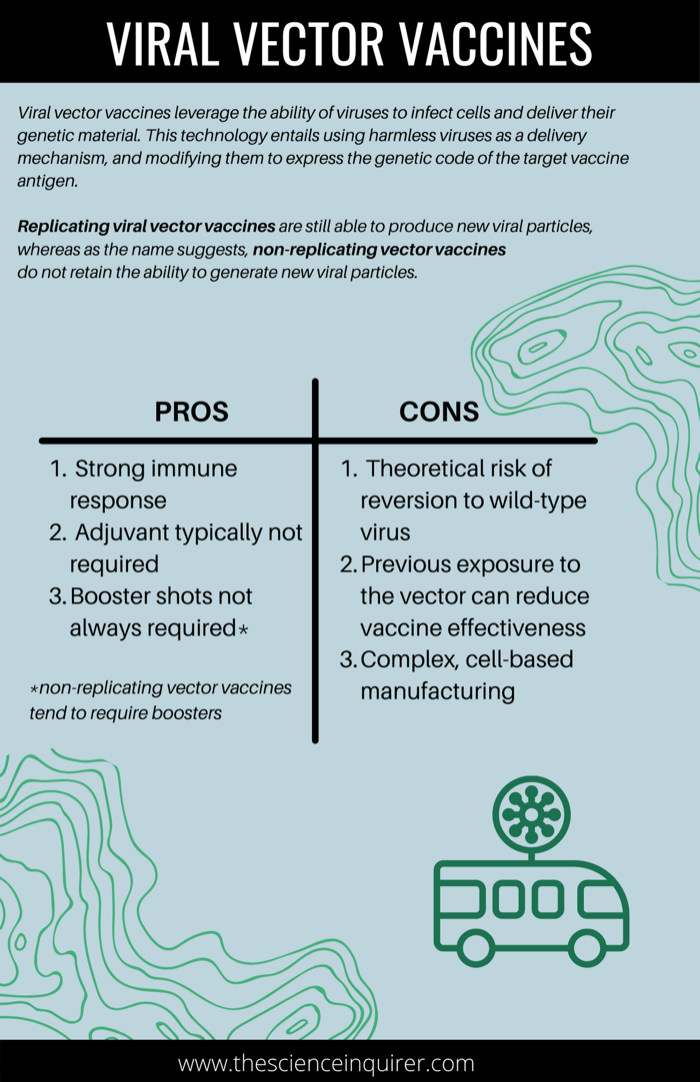
5. Nucleic Acid Vaccines
Nucleic acid vaccines are different from their other vaccine counterparts in that they do not deliver protein antigen to cells and tissues 2. Instead, they deliver a type of genetic code, in the form of either DNA or RNA, which are necessary to make protein antigens. The cells of the body are then able to produce the protein antigen themselves, thereby stimulating an immune response.
There are 2 sub-types of nucleic acid vaccine, but first....
What is the difference between RNA and DNA?
First, it’s important to distinguish between DNA and messenger RNA (mRNA). DNA is a double-stranded molecule that contains the biological instructions that make each individual unique. These instructions actually code for proteins. Most DNA is housed in a part of the cell referred to as the nucleus. The conversion of DNA to essential proteins first requires DNA to be converted to a single-stranded intermediate, known as mRNA. Put simply, mRNA can be thought of as the blueprint needed to make protein. This conversion from DNA to mRNA is necessary because only messenger RNA can be transported to the appropriate location, a sort of “protein factory” in the cytoplasm of a cell, where proteins can be synthesized.
To simplify this, imagine a chef wants to bake a very special chocolate cake and the desired recipe is in a library.44 Think of DNA as a large cook book in a library, and think of the library as the nucleus. The cook book contains thousands of recipes.44 At this library, you cannot check books out so the chef has to make a photocopy of the cake recipe. This photocopy of the recipe is the mRNA and can now be transported to the kitchen (the cytoplasm, aka the protein-making factory), where the chef will replicate this recipe to bake the very special chocolate cake (the protein).44
A brief history on nucleic acid vaccines
While many believe that nucleic acid (RNA and DNA) vaccines are a new concept sparked by the COVID-19 pandemic, the origins of these vaccines can be traced back to the 90s.38,39 In 1997, DNA vaccines were proven effective at protecting two chimpanzees from contracting AIDS.40 Unfortunately, as biomedical research often goes, the first human clinical trials of nucleic acid vaccines flopped because humans didn’t mount the immune response necessary to confer protection against disease.41 Ultimately, the early phase clinical trials launched by companies failed and the hype surrounding nucleic acid vaccines fizzled out.41 The primary challenge which needed to be overcome was how to get DNA or RNA into human cells.41 Due to their high negative charge, nucleic acids struggle to cross the cell membrane.
While industry interest initially diminished, scientists continued to conduct research into DNA and RNA vaccines and any mechanisms that could facilitate the entry of nucleic acids into cells and enhance their safety profile. In the two decades leading up to the COVID-19 pandemic, technical improvements led to the resurgence of interest in nucleic acid vaccines, ranging from more effective delivery approaches to new formulations and immune adjuvants 42, 43.
This preliminary research laid the groundwork for what would become the fastest vaccine to go from development to deployment in history.
a) mRNA Vaccines
An mRNA vaccine works by deliberately introducing mRNA, a single-stranded genetic sequence, inside a lipid (fat) membrane into target cells to produce viral proteins.2 In other words, unlike whole pathogen or vector vaccines, there is no virus or viral fragment in the vaccine. Once inside the cell, it sets up a short-term protein factory outside the nucleus in the cytoplasm (the factory), attaches to ribosomes (the factory workers), and the mRNA acts as the template that allows the ribosomes to translate the mRNA into protein.45 Within a day, the mRNA is degraded and the synthesized proteins are what actually function as the drug or vaccine.45
How does mRNA enter the cell?
As mentioned previously, nucleic acids struggle to cross cell membranes. Due to the chemistry of mRNA, it is less “stable” than DNA, meaning it breaks down easily.46 In natural physiological terms, this is valuable because RNA is generally a short-term molecule that cells degrades when it is no longer needed, whereas DNA needs to be more stable as it is the ultimate carrier of our genetic information. To combat how easily mRNA breaks down, RNA vaccines use mRNA inside a lipid (fat) membrane, otherwise referred to as a “lipid nanoparticle”.45 This fatty membrane, or lipid nanoparticle, protects the mRNA as it enters the body and helps it enter cells by merging with the host cell membrane.45
These types of vaccines have been used to create immunity against the following diseases: At present, there are no licensed RNA vaccines but there are many in development.
These are the COVID-19 mRNA vaccines currently in the development pipeline, or authorized for emergency use:
1. The Moderna COVID-19 Vaccine (mRNA-1273)
2. The Pfizer-BioNTech Vaccine (BNT 162b2)
3. CVnCoV, CureVac's mRNA-based vaccine candidate
What are the advantages of mRNA vaccines?
- Non-infectious: mRNA vaccines contain genetic, not viral, material. As such, mRNA is non-infectious and cannot revert to the virulent disease. There is no potential risk of infection.47
- Non-integrating: Unlike DNA which can integrate unpredictably into the genome, mRNA from a vaccine does not affect or interact with a person’s DNA because it cannot enter the nucleus of cells. As such, mRNA is non-integrating. In other words, there is no risk of an mRNA vaccine causing DNA mutations (i.e. insertional mutagenesis).47
- Natural degradation: mRNA is naturally broken down by normal cellular processes47
- No issues with anti-vector immunity: Unlike viral vector vaccines, mRNA vaccines do not utilize viral vectors as a delivery mechanism. As such, mRNA vaccines can be repeatedly administered, if necessary.47
- Positive trial results: Clinical trial results thus far indicate that these vaccines generate a reliable immune response and are well-tolerated by healthy individuals with few side effects. The most common adverse events reported in the Pfizer BioNtech mRNA vaccine during clinical trials in participants over the age of 16 included pain at the site of injection, fatigue, headache, muscle pain, joint pain, fever,, nausea, and malaise. 48
- Cell-free manufacturing: Manufacturing does not require any cells or animal substrates which dramatically reduces the risk of contamination and ensures high batch-to-batch consistency.43
- Rapid and scalable manufacturing: The technology associated with producing mRNA vaccines can bypass traditionally time-consuming processes allowing for highly standardized commercial manufacturing.47
What are the disadvantages of mRNA vaccines?
- “Naked” RNA is a highly unstable molecule requiring a carrier molecule speculated to cause allergic reactions in some individuals: The ubiquitous nature of enzymes which quickly degrade RNA requires mRNA in vaccines to contain a “carrier” to allow it to safely cross the cellular plasma membrane. In the case of current mRNA vaccines authorized for emergency use during the COVID-19 pandemic, lipid nanoparticles are the carrier of choice.49 When complexed with these positively-charged lipid nanoparticles, mRNA becomes more resistant to enzyme degradation.49 This is listed as a disadvantage because some individuals have experienced strong allergic reactions (i.e. anaphylaxis) to the Pfizer BioNTech and Moderna mRNA vaccines. Those with a history of severe allergic reactions to any vaccine component should refrain from receiving the vaccine until consulting with their physician. Scientists have speculated that this may be due to polyethylene glycol (PEG), one of the lipid components which make up the lipid nanoparticle “carrier” discussed above.50 While a growing body of evidence suggests that PEG may be the culprit, consensus among the medical and scientific community has yet to be reached. It is, however, important to note that both Pfizer and Moderna have acknowledged that PEG has the ability to cause allergic reactions.48, 50
- Lower immunogenicity: By encoding only fragments of the virus, its ability to stimulate a robust immune response relative to LA vaccines is limited.43
- Freezing is required: While many conventional vaccines require refrigeration, RNA vaccines must be frozen to maintain stability. The Pfizer vaccine requires storage in an ultra-cold freezer between -80⁰C and -60⁰C, whereas the Moderna vaccine requires storage between 25°C and -15°C. 51,52 The discrepancy in temperatures between the two vaccines has to do with the formulation. Moderna’s lipid nanoparticle composition has particular properties and structures which allow the vaccine to remain stable in somewhat warmer conditions relative to the Pfizer vaccine.53
- Several unknowns: This includes antigen dependent enhancement (ADE), the length of immunity, whether the vaccine can prevent asymptomatic transmission, effectiveness in babies and infants, and the safety profile in pregnant women.
b) DNA Vaccines
A DNA vaccine is a type of vaccine that deliberately introduces DNA, a double-stranded genetic code, into target cells where the code is translated into an antigen protein.2 The term DNA vaccine is a somewhat of a misnomer in that DNA is not used as an antigen. The DNA encodes for the antigen that is expressed in host cells. Typically, a bacterial plasmid (definition: a small circular piece of DNA from a bacterial cell that is independent of its chromosomal DNA) is used and a gene coding for the antigen against which an immune response is sought is inserted.68 In other words, the code for a foreign antigen is inserted into the bacterial genome.
Similar to RNA vaccines, there is no virus or viral fragment present in a DNA vaccine. Unlike mRNA, DNA is more stable so it does not necessarily require protection within a lipid nanoparticle. That being said, DNA typically needs assistance crossing cell membranes.
How does DNA enter the cell?
Typically, a process known as “electroporation” is administered with a DNA vaccine.2 This technique utilizes low level electronic current to help the bodies’ cells take up the DNA vaccine. DNA is then converted to mRNA inside the cell nucleus (or the library in our previous analogy) and transported to the cytoplasm (the protein-making factory). Only once it is mRNA can the body convert this to protein antigens which will be recognized by the body to stimulate an immune response.2
These types of vaccines have been used to create immunity against the following diseases: At present, there are no licensed DNA vaccines, but there are many in development.
COVID-19 DNA vaccines in the development pipeline:
1. INO-4800, Inovio's DNA Vaccine Candidate
2. GX-19N, Genexine's DNA Vaccine Candidate
3. ZyCoV-D, Zydus cadila's DNA Vaccine Candidate
What are the advantages of DNA vaccines?
- Long-term persistence of immunogenicity: DNA vaccines express antigen for a long period of time which triggers immunological memory. Not only is the immune response long-lasting, but it also mimics natural infection. 68
- Non-virulent: Plasmids do not replicate, therefore DNA vaccines cannot cause disease. 68
- Focused immune response: The immune response is focused on a specific antigen of interest, as oppose to the entire virus. 68
- Stability of vaccine for storage and shipping: DNA is a stable molecule which can be stored at room temperature in both solution or dried form. Conventional vaccines require specialized refrigerators. 68
- DNA sequences (and thus antigen) can easily be modified: If the need arises, DNA sequences alterations can easily be introduced.68
- Easy to manufacture in large amounts68
What are the disadvantages of DNA vaccines?
- Potential integration into host genome (i.e. insertional mutagenesis): Injected DNA can be taken up by cells and integrated into host’s cellular genetic material as DNA needs to reach the nucleus to be decoded.49 This may cause the cell to become cancerous. 68
- Potential for impurities must be rigorously controlled during the manufacturing process: Data must be provided on any contaminants present once plasmids are purified.69
- Autoimmune reactions and the Induction of anti-DNA antibodies: DNA vaccines may prompt the formation of antibodies against injected foreign DNA which can trigger an undesired autoimmune reaction. 68 More specifically, bacterial DNA can trigger IgG anti-DNA autoantibodies. These types of antibodies are often associated with auto-immune glomerulonephritis in diseases like systemic lupus.69 However, scientific advancements targeting the effectiveness of DNA delivery and dosage may ameliorate this concern.69
- DNA vaccines are limited to the formation of immune responses against protein antigens: As anticipated, DNA vaccines cannot be formed against polysaccharide (complex sugar) antigens. 68
- Concerns over developmental and reproductive toxicity: DNA vaccines are typically contraindicated for pregnant women. A study showed that when DNA was injected into pregnant mice, DNA can reach the fetus. If DNA is taken up by fetal cells, this can induce something known as “immunological tolerance” in the progeny.70 Tolerance leads to increased susceptibility to infection. There are several potential solutions to this including utilizing a promoter (definition: a sequence of DNA required to turn a gene on or off) that is muscle-specific, and inactive during early pre-natal and early post-natal life.70
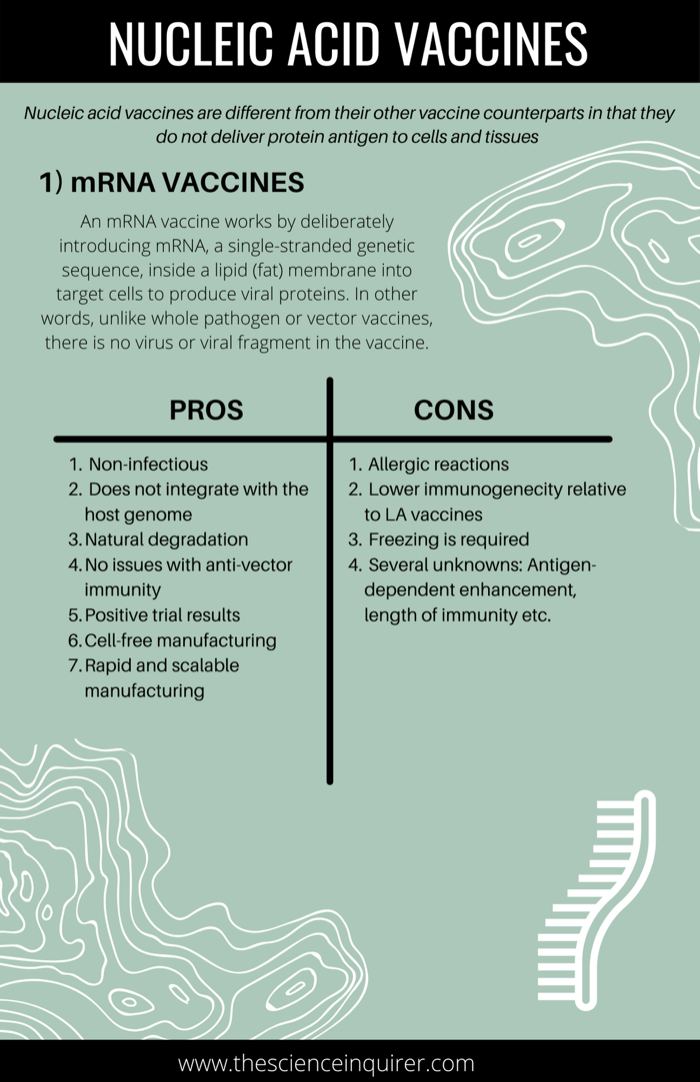
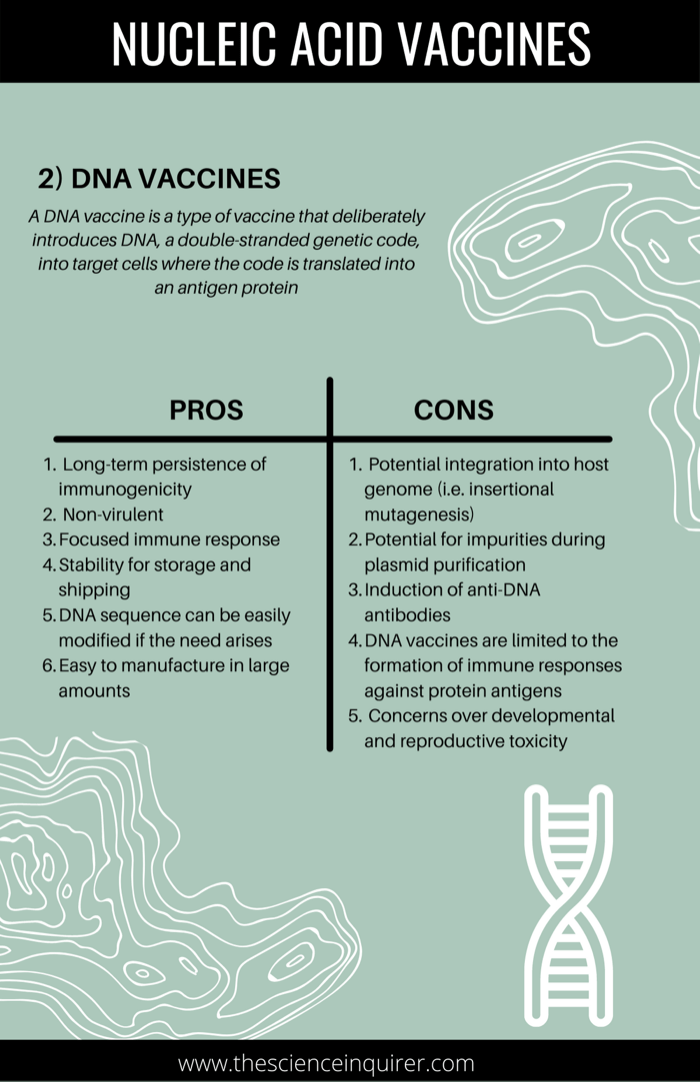
Reference List
1. Mak TW, Maya Rani Chaddah, Mary Evelyn Saunders. The Immune Response : Basic and Clinical Principles. Elsevier, C; 2006:695-749. https://www.sciencedirect.com/book/9780120884513/the-immune-response
2. Types of vaccine | Vaccine Knowledge. Ox.ac.uk. Published 2019. https://vk.ovg.ox.ac.uk/vk/types-of-vaccine
3. Lee S, Nguyen MT. Recent Advances of Vaccine Adjuvants for Infectious Diseases. Immune Network. 2015;15(2):51. doi:10.4110/in.2015.15.2.51
4. Lauring AS, Jones JO, Andino R. Rationalizing the development of live attenuated virus vaccines. Nature Biotechnology. 2010;28(6):573-579. doi:10.1038/nbt.1635
5. Sharma LB, Ohgimoto S, Kato S, et al. Contribution of Matrix, Fusion, Hemagglutinin, and Large Protein Genes of the CAM-70 Measles Virus Vaccine Strain to Efficient Growth in Chicken Embryonic Fibroblasts. Journal of Virology. 2009;83(22):11645-11654. doi:10.1128/jvi.01110-09
6. Adjuvants help vaccines work better. Published 2019. https://www.cdc.gov/vaccinesafety/concerns/adjuvants.html
7. Verma AS, Anchal Singh. Animal Biotechnology : Models in Discovery and Translation. Academic Press; 2020.
8. Keller-Stanislawski B, Englund JA, Kang G, et al. Safety of immunization during pregnancy: A review of the evidence of selected inactivated and live attenuated vaccines. Vaccine. 2014;32(52):7057-7064. doi:10.1016/j.vaccine.2014.09.052
9. Keystone JS, Kozarsky PE, Connor BA, Nothdurft HD, Mendelson M, Leder K. Travel Medicine. Elsevier; 2019.
10. Burrell CJ, Howard CR, Murphy FA. Fenner and White’s Medical Virology. Elsevier Science Publishing Co Inc, Boston; 2017.
11. Sanders B, Koldijk M, Schuitemaker H. Inactivated Viral Vaccines. Vaccine Analysis: Strategies, Principles, and Control. 2014;45-80. Published 2014 Nov 28. doi:10.1007/978-3-662-45024-6_2
12. Louten J, Reynolds N. Essential Human Virology. Elsevier; 2016.
13. Vaccines: Vac-Gen/Additives in Vaccines Fact Sheet. Centers for Disease Control & Prevention. Published 2019. https://www.cdc.gov/vaccines/vac-gen/additives.htm
14. The Children’s Hospital of Philadelphia. Vaccine Ingredients – Formaldehyde | Children’s Hospital of Philadelphia. Chop.edu. Published November 6, 2014. https://www.chop.edu/centers-programs/vaccine-education-center/vaccine-ingredients/formaldehyde
15. Tulpule K, Dringen R. Formaldehyde in brain: an overlooked player in neurodegeneration? Journal of Neurochemistry. 2013;127(1):7-21. doi:10.1111/jnc.12356
16. Burgos-Barragan G, Wit N, Meiser J, et al. Mammals divert endogenous genotoxic formaldehyde into one-carbon metabolism. Nature. 2017;548(7669):549-554. doi:10.1038/nature23481
17. European Food Safety Authority. Endogenous formaldehyde turnover in humans compared with exogenous contribution from food sources. EFSA Journal. 2014;12(2). doi:https://doi.org/10.2903/j.efsa.2014.3550
18. Bosetti C, McLaughlin JK, Tarone RE, Pira E, La Vecchia C. Formaldehyde and cancer risk: a quantitative review of cohort studies through 2006. Annals of Oncology. 2008;19(1):29-43. doi:10.1093/annonc/mdm202
19. Moyle PM, Toth I. Modern Subunit Vaccines: Development, Components, and Research Opportunities. ChemMedChem. 2013;8(3):360-376. doi:10.1002/cmdc.201200487
20. Recombinant vaccine - Latest research and news | Nature. Nature.com. Published August 13, 2019. https://www.nature.com/subjects/recombinant-vaccine
21. Center for Biologics Evaluation and Research. Biomarkers of Vaccine S&E for Encapsulated Bacterial Pathogens. U.S. Food and Drug Administration. Published 2018. https://www.fda.gov/vaccines-blood-biologics/biologics-research-projects/biomarkers-vaccine-safety-and-effectiveness-encapsulated-bacterial-pathogens
22. CDC/NCIRD. Immunology and Vaccine-Preventable Diseases – Pink Book – Principles of Vaccination.; 2015. https://www.cdc.gov/vaccines/pubs/pinkbook/downloads/prinvac.pdf
23. CDC/NCIRD. Understanding How Vaccines Work.; 2018. https://www.cdc.gov/vaccines/hcp/conversations/downloads/vacsafe-understand-color-office.pdf
24. Noad R, Roy P. Virus-like particles as immunogens. Trends in Microbiology. 2003;11(9):438-444. doi:10.1016/s0966-842x(03)00208-7
25. Mohsen M, Gomes A, Vogel M, Bachmann M. Interaction of Viral Capsid-Derived Virus-Like Particles (VLPs) with the Innate Immune System. Vaccines. 2018;6(3):37. doi:10.3390/vaccines6030037
26. Urakami A, Sakurai A, Ishikawa M, et al. Development of a Novel Virus-Like Particle Vaccine Platform That Mimics the Immature Form of Alphavirus. Staats HF, ed. Clinical and Vaccine Immunology. 2017;24(7). doi:10.1128/cvi.00090-17
27. Qing G, Gong N, Chen X, et al. Natural and engineered bacterial outer membrane vesicles. Biophysics Reports. 2019;5(4):184-198. doi:10.1007/s41048-019-00095-6
28. Mancini F, Rossi O, Necchi F, Micoli F. OMV Vaccines and the Role of TLR Agonists in Immune Response. International Journal of Molecular Sciences. 2020;21(12):4416. doi:10.3390/ijms21124416
29. Tan K, Li R, Huang X, Liu Q. Outer Membrane Vesicles: Current Status and Future Direction of These Novel Vaccine Adjuvants. Frontiers in Microbiology. 2018;9(9). doi:10.3389/fmicb.2018.00783
30.MODULE 2 – Toxoid vaccines - WHO Vaccine Safety Basics. Vaccine-safety-training.org. Published 2019. https://vaccine-safety-training.org/toxoid-vaccines.html
31. Hunter JE, Ramos L, Wolfe JH. Viral Vectors in the CNS. Reference Module in Neuroscience and Biobehavioral Psychology, Elsevier. Published online 2017. doi:https://doi.org/10.1016/B978-0-12-809324-5.02446-9
32. Robert-Guroff M. Replicating and non-replicating viral vectors for vaccine development. Current Opinion in Biotechnology. 2007;18(6):546-556. doi:10.1016/j.copbio.2007.10.010
33. van Riel D, de Wit E. Next-generation vaccine platforms for COVID-19. Nature Materials. 2020;19(8):810-812. doi:10.1038/s41563-020-0746-0
34. Rauch S, Jasny E, Schmidt KE, Petsch B. New Vaccine Technologies to Combat Outbreak Situations. Frontiers in Immunology. 2018;9. doi:10.3389/fimmu.2018.01963
35. Venkatraman N, Anagnostou N, Bliss C, et al. Safety and immunogenicity of heterologous prime-boost immunization with viral-vectored malaria vaccines adjuvanted with Matrix-MTM. Vaccine. 2017;35(45):6208-6217. doi:10.1016/j.vaccine.2017.09.028
36. Ulmer JB, Mason PW, Geall A, Mandl CW. RNA-based vaccines. Vaccine. 2012;30(30):4414-4418. doi:10.1016/j.vaccine.2012.04.060
37. What are viral vector-based vaccines and how could they be used against COVID-19? www.gavi.org. Accessed February 2, 2021. https://www.gavi.org/vaccineswork/what-are-viral-vector-based-vaccines-and-how-could-they-be-used-against-covid-19
38. Tang D, DeVit M, Johnston SA. Genetic immunization is a simple method for eliciting an immune response. Nature. 1992;356(6365):152-154. doi:10.1038/356152a0
39. Ying H, Zaks T, Wang R. Cancer therapy using a self-replicating RNA vaccine. Nature Med. 1999;5(7):823-827. doi:10.1038/10548
40. Altman LK. Vaccine Protects Two Chimps From AIDS (Published 1997). The New York Times. https://www.nytimes.com/1997/04/30/us/vaccine-protects-two-chimps-from-aids.html. Published April 30, 1997. Accessed January 18, 2021.
41. Mullin E. Vaccine Tech 30 Years in the Making Is Getting Put to the Ultimate Test. Medium. Published December 18, 2020. Accessed January 18, 2021. https://futurehuman.medium.com/vaccine-tech-30-years-in-the-making-is-getting-put-to-the-ultimate-test-3e5607257db0
42. Kutzler MA, Weiner DB. DNA vaccines: ready for prime time? Nature Reviews Genetics. 2008;9(10):776-788. doi:10.1038/nrg2432
43. Zhang C, Maruggi G, Shan H, Li J. Advances in mRNA Vaccines for Infectious Diseases. Frontiers in Immunology. 2019;10(594). doi:10.3389/fimmu.2019.00594
44. Hubaud A. RNA vaccines: a novel technology to prevent and treat disease. Science in the News. Published May 5, 2015. http://sitn.hms.harvard.edu/flash/2015/rna-vaccines-a-novel-technology-to-prevent-and-treat-disease/
45. ServickMar. 25 K, 2020, Pm 12:20. Meet the company that has just begun testing a coronavirus vaccine in the United States. Science | AAAS. Published March 25, 2020. https://www.sciencemag.org/news/2017/02/mysterious-2-billion-biotech-revealing-secrets-behind-its-new-drugs-and-vaccines
46. EMBL-EBI. RNA | Biomacromolecular structures. Accessed January 19, 2021. https://www.ebi.ac.uk/training-beta/online/courses/biomacromolecular-structures/rna/
47. Pardi N, Hogan MJ, Porter FW, Weissman D. mRNA vaccines — a new era in vaccinology. Nature Reviews Drug Discovery. 2018;17(4):261-279. doi:10.1038/nrd.2017.243
48. Strategic Advisory Group of Experts (SAGE) Working Group on COVID-19 Vaccines, World Health Organization (WHO). Background Document on the MRNA Vaccine BNT162b2 (Pfizer-BioNTech) against COVID-19.; 2021.
49. Nanomedicine and the COVID-19 vaccines. Nature Nanotechnology. Published online November 27, 2020. doi:10.1038/s41565-020-00820-0
50.de Vrieze J. Suspicions grow that nanoparticles in Pfizer’s COVID-19 vaccine trigger rare allergic reactions. Science | AAAS. Published December 21, 2020. https://www.sciencemag.org/news/2020/12/suspicions-grow-nanoparticles-pfizer-s-covid-19-vaccine-trigger-rare-allergic-reactions
51. Moderna COVID-19 Vaccine Information | CDC. www.cdc.gov. Published December 22, 2020. https://www.cdc.gov/vaccines/covid-19/info-by-product/moderna/index.html
52. Pfizer-BioNTech COVID-19 Vaccine Information | CDC. www.cdc.gov. Published December 19, 2020. https://www.cdc.gov/vaccines/covid-19/info-by-product/pfizer/index.html
53. Simmons-Duffin S. Why Does Pfizer’s COVID-19 Vaccine Need To Be Kept Colder Than Antarctica? NPR.org. Published November 17, 2020. https://www.npr.org/sections/health-shots/2020/11/17/935563377/why-does-pfizers-covid-19-vaccine-need-to-be-kept-colder-than-antarctica
54. Lowe D, 2020. Antibody-Dependent Enhancement. In the Pipeline. Published December 18, 2020. Accessed February 9, 2021. https://blogs.sciencemag.org/pipeline/archives/2020/12/18/antibody-dependent-enhancement
55. Garber K. Coronavirus vaccine developers wary of errant antibodies. Nature Biotechnology. Published online June 5, 2020. doi:10.1038/d41587-020-00016-w
56. KIM HW, CANCHOLA JG, BRANDT CD, et al. RESPIRATORY SYNCYTIAL VIRUS DISEASE IN INFANTS DESPITE PRIOR ADMINISTRATION OF ANTIGENIC INACTIVATED VACCINE12. American Journal of Epidemiology. 1969;89(4):422-434. doi:10.1093/oxfordjournals.aje.a120955
57. Lee WS, Wheatley AK, Kent SJ, DeKosky BJ. Antibody-dependent enhancement and SARS-CoV-2 vaccines and therapies. Nature Microbiology. 2020;5(10):1185-1191. doi:10.1038/s41564-020-00789-5
58. A Phase 1/2/3 Study to Evaluate the Safety, Tolerability, Immunogenicity, and Efficacy of RNA Vaccine Candidates against COVID-19 in Healthy Individuals. Pfizer; 2020:29. Accessed January 20, 2021. https://pfe-pfizercom-d8-prod.s3.amazonaws.com/2020-11/C4591001_Clinical_Protocol_Nov2020.pdf
59. A Phase 3, Randomized, Stratified, Observer-Blind, Placebo-Controlled Study to Evaluate the Efficacy, Safety, and Immunogenicity of MRNA-1273 SARS-CoV-2 Vaccine in Adults Aged 18 Years and Older.; 2020:41.
60. Commissioner O of the. Pfizer-BioNTech COVID-19 Vaccine Frequently Asked Questions. FDA. Published online January 6, 2021. https://www.fda.gov/emergency-preparedness-and-response/mcm-legal-regulatory-and-policy-framework/pfizer-biontech-covid-19-vaccine-frequently-asked-questions
61. Pfizer and BioNTech Announce Publication of Results from Landmark Phase 3 Trial of BNT162b2 COVID-19 Vaccine Candidate in The New England Journal of Medicine | Pfizer. Pfizer.com. Published 2020. https://www.pfizer.com/news/press-release/press-release-detail/pfizer-and-biontech-announce-publication-results-landmark
62. Polack FP, Thomas SJ, Kitchin N, et al. Safety and Efficacy of the BNT162b2 mRNA Covid-19 Vaccine. New England Journal of Medicine. 2020;383(27). doi:10.1056/nejmoa2034577
63. Aziz S. How long will the COVID-19 vaccine protect you? Here’s what we know so far. Global News. https://globalnews.ca/news/7523595/coronavirus-vaccine-protection-immunity-covid-19/
64. Saey TH, Lambert J. Here are answers to 6 burning questions about COVID-19 vaccines. Science News. Published December 8, 2020. https://www.sciencenews.org/article/covid-19-coronavirus-vaccines-questions-social-distance-mask-transmission
65. Bell’s palsy - Symptoms and causes. Mayo Clinic. Published 2018. https://www.mayoclinic.org/diseases-conditions/bells-palsy/symptoms-causes/syc-20370028
66. Mutsch M, Zhou W, Rhodes P, et al. Use of the Inactivated Intranasal Influenza Vaccine and the Risk of Bell’s Palsy in Switzerland. New England Journal of Medicine. 2004;350(9):896-903. doi:10.1056/nejmoa030595
67. The Facts About Pfizer and BioNTech’s COVID-19 Vaccine | Pfizer. Pfizer.com. Published January 6, 2021. Accessed February 9, 2021. https://www.pfizer.com/news/hot-topics/the_facts_about_pfizer_and_biontech_s_covid_19_vaccine#_edn8
68. Fahim Halim Khan. The Elements of Immunology. Pearson Education, Cop; 2009.
69. World Health Organization. Guidelines for Assuring the Quality and Nonclinical Safety Evaluation of DNA Vaccines.; 2007. https://www.who.int/biologicals/publications/trs/areas/vaccines/dna/Annex%201_DNA%20vaccines.pdf?ua=1
70. Górecki DC, Simons JP. The dangers of DNA vaccination. Nature Medicine. 1999;5(2):126-126. doi:10.1038/5473
71. HIGHLIGHTS of PRESCRIBING INFORMATION: ERVEBO® (Ebola Zaire Vaccine, Live) Suspension for Intramuscular Inj Ection Initial U.S. Approv Al: 2019.; 2019:6. Accessed February 16, 2021. https://www.fda.gov/media/133748/download
72. Johnson & Johnson Announces European Commission Approval for Janssen’s Preventive Ebola Vaccine | Johnson & Johnson. Content Lab U.S. Accessed February 16, 2021. https://www.jnj.com/johnson-johnson-announces-european-commission-approval-for-janssens-preventive-ebola-vaccine
73. Ramasamy MN, Minassian AM, Ewer KJ, et al. Safety and immunogenicity of ChAdOx1 nCoV-19 vaccine administered in a prime-boost regimen in young and old adults (COV002): a single-blind, randomised, controlled, phase 2/3 trial. The Lancet. 2020;0(0). doi:10.1016/S0140-6736(20)32466-1
74. COVID-19. Codagenix. Accessed February 17, 2021. https://codagenix.com/vaccine-programs/covid-19/
75. Layt S. Griffith University partners with Indian firm for COVID-19 vaccine. Brisbane Times. Published September 3, 2020. Accessed February 17, 2021. https://www.brisbanetimes.com.au/national/queensland/griffith-university-partners-with-indian-firm-for-covid-19-vaccine-20200903-p55s65.html
76. Poland GA, Ovsyannikova IG, Kennedy RB. SARS-CoV-2 immunity: review and applications to phase 3 vaccine candidates. The Lancet. Published online October 2020. doi:10.1016/s0140-6736(20)32137-1
77. Corum J, Zimmer C. How the Sinopharm Vaccine Works. The New York Times. https://www.nytimes.com/interactive/2020/health/sinopharm-covid-19-vaccine.html. Accessed February 17, 2021.
78. COVAXIN - India’s First Indigenous Covid-19 Vaccine | Bharat Biotech. www.bharatbiotech.com. https://www.bharatbiotech.com/covaxin.html
79. Novavax COVID-19 Vaccine Demonstrates 89.3% Efficacy in UK Phase 3 Trial | Novavax Inc. - IR Site. ir.novavax.com. https://ir.novavax.com/news-releases/news-release-details/novavax-covid-19-vaccine-demonstrates-893-efficacy-uk-phase-3
80. Home. website. Accessed February 17, 2021. https://www.adaptvac.com
81. Bavarian Nordic and AdaptVac finalizes license deal on COVID-19 vaccine (OTCMKTS:BVNRY). SeekingAlpha. Accessed February 17, 2021. https://seekingalpha.com/news/3593452-bavarian-nordic-and-adaptvac-finalizes-license-deal-on-covidminus-19-vaccine
82. Jones I, Roy P. Sputnik V COVID-19 vaccine candidate appears safe and effective. The Lancet. 2021;0(0). doi:10.1016/S0140-6736(21)00191-4
83. Sadoff J, Le Gars M, Shukarev G, et al. Interim Results of a Phase 1–2a Trial of Ad26.COV2.S Covid-19 Vaccine. New England Journal of Medicine. Published online January 13, 2021. doi:10.1056/nejmoa2034201